The eBay Oils (Part 2)
Welcome back to the eBay oils! If you missed the first installment of this article, you can read it here.
True to his word, Ryan listened to his Passat (if you’ll recall, it was making a noise that sounded like “Sell Me” in German) and got his wife a new Hyundai Elantra this month. Free oil changes were not included as part of the deal, so Ryan will be changing his Elantra’s oil himself. And while he’s willing to experiment with his 1984 ½ ton Chevy pickup truck, it’s doubtful (for now, at least), that he’ll be using either Wolf’s Head or Fox Head oil in his new car.
Wolf’s Head SD 10W/40
Wolf’s Head Motor Oil Company was originally founded in Pennsylvania in 1879 as “Pennsylvania Crude.” (The source of this is Wikipedia, so take this information with a grain of salt.) I’d place the can from the late-1970s or early 1980s. It is “Formulated from Finest Quality Base Stocks and Superior Motor Oil Additives” and back then, like today, the container gives no actual clue as to what the additives are. That’s where we come in. The report is an interesting one. Note the lack of calcium, which is used in almost all engine oils nowadays as a detergent/dispersant additive. Instead of calcium, it contains a lot of copper as an additive. This is a trend we were glad to see die. All that copper in the oil masks bronze wear.
Wolf’s Head ATF
Wolf’s Head also made (makes?) an ATF, though the only selling point on this can is that it’s “Refinery Sealed.” Maybe they couldn’t think of anything interesting to say about it, because honestly it looks just like a lot of ATFs we see today: mostly phosphorus and zinc, with a smattering of other additives thrown in for good measure.
Fox Head 40W
Oil marketing has come a long way since the days of yore. Today when you want to buy a high-dollar oil you’ll find it has a name that conjures up something special: speed (Red Line), richness (Royal Purple), slickness (Amsoil), racing (Mobil 1), space-age (Quantum Blue), etc. I’m not sure what “Elf” is meant to conjure up, but they follow it up with “Excellium” so you know it’s Excellent oil. Contrast those names with our next contender, Fox Head, which just makes me think of…well, a fox head. In my oil. Fortunately, that’s not what analysis turned up. (Although…we don’t test specifically for fox heads, so we could be missing something here.) The Fox Head can is red, white, and blue, so you can feel patriotic when you buy it (unless
you’re in Canada. Then you can indulge in justified rage about Americans and how we think we’re the center of the world). Fox Head oil was made by the Tritex Petroleum company out of Brooklyn, NY, and my extensive research (aka first-page Google results) tells me the company still exists and is presently located in Tulsa, Oklahoma. The logo, a sly-looking fox, has nothing on today’s slick oil packages. And the oil itself also has nothing on today’s oils: the oil itself is nearly bereft of additives. Basically a mineral oil, it has a little magnesium, phosphorus, and zinc in it, and not a lot else (Figure 3). This is not necessarily a problem, however. As you’ll recall in the article when Ryan used 30W aircraft oil in his truck, wear went up a little but the engine didn’t fail or anything. Still, I won’t be putting it in my Outback anytime soon.
Shell Rotella S 10W
Shell Rotella has been around for a long time. It’s good oil, and since they’ve been making it for decades, they’ve pretty much got the routine down and haven’t messed with it a lot over the years. Unlike now, when you can actually follow Shell Rotella on Twitter (who knew Rotella had so much to say?), back in the day Rotella had to get by just by on traditional advertising and word of mouth. We called Shell to see how long they’ve been making this oil and the guy not only could not tell me, but he was unable to tell me who might know. Surely someone at that company has a historical file? If so, they’re not sharing that info with plebeians like us. He did mention that Rotella really made its name in the ’70s, though I’m guessing this can of SF, SE, SC oil was made in the late ’80s. He also said the “S” versions of Rotella were sold internationally, and indeed, this can came from our friendly neighbors to the north (*waves hi to Canada). Suffice it to say that the oil has changed very little over the years. Its main additives are the same as what we see today, but the interesting part of this oil is that it’s a 10W (Figure 4). We often see heavy-duty thin-grade additive packages in tractor-hydraulic fluids, which are used in systems like transmissions and hydraulic systems in off-highway equipment like bulldozers and backhoes. Note the TBN of this oil read higher than most of the others we’re talking about. That’s because of the high calcium level¾the TBN is based on the level of calcium sulfinate and/or magnesium sulfinate. When those compounds aren’t present, you get a low TBN.
Quaker State 30W & 10W
Next up is another oil familiar to today’s buyers: Quaker State. This HDX oil is a straight 30W, and Quaker State was ahead of the marketing game on this one. The can’s copy touts their “high quality,” “modern refineries,” and “quality control laboratories,” and this was about as scientific as it got 30 or 40 years ago. The additive package is fairly stout, though like Wolf’s Head oil, calcium is in short supply (Figure 5). We also bought a can of Quaker State 10W oil, and when we looked at the report we had to go back and double-check the can to make sure this wasn’t actually ATF. It’s not: it’s labeled as an SAE 10W oil, though the additive package looks an awful lot like what we see of out today’s transmission oil. Interestingly, there’s a lot of barium in it (Figure 6). Barium must be expensive, or else it doesn’t do much, because we rarely see it in oil samples of any type nowadays. This is engine oil, but it might work in transmissions too.
Quaker State Deluxe 10W/40 & Sterling 10W/40 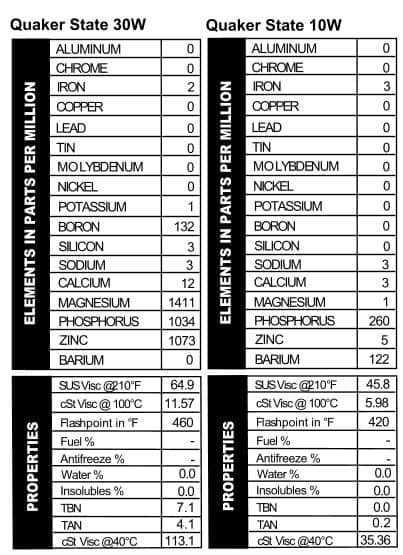
Apparently we are fans of Quaker State, because we also tested a couple more varieties: Quaker State Deluxe, and Quaker State Sterling. As you know, modern oil companies generally have a lot of different brands under the same name (for example, Valvoline has TK, TK, and TK, as well as TK). For the most part, these oils are mostly the same; they’ll throw in a few slight differences in additives and call it good. These cans of Quaker State, however, were mostly pretty different. The Deluxe version looked a lot like their 30W oil (but more calcium–Figure 7). Quaker State Sterling HD 10W/40, on the other hand, went out on a limb with almost 800 ppm sodium, almost no magnesium, and then levels of calcium, phosphorus, and zinc that are comparable with today’s oils. Touted as “Energy Saving Motor Oil,” Quaker State was getting its game on in pushing this brand: it mentions “special friction modifying additives,” the longevity of the company (over 60 years when the can was made), and its suitability for those wishing to follow extended drain intervals. Heck, I’m sold, and I see this stuff all the time.
Mobil Special 10W/30 & Artic 20-20W
Mobil is no slouch in the marketing department, but they really outdid themselves with the can we tested, “Mobil Special.” The name alone tells you all you need to know about why to buy this oil. All oil companies like to mess with their additive packages, and Mobil, like the others, changes their oil up fairly frequently. That was the case back in the day too, because the additive package in this “Special” oil is different from what we typically see in today’s oil. Apparently Mobil was an early rider on the ZDDP train, because this oil is chock-full of both phosphorus and zinc. Calcium and magnesium are present too, but at lower levels (Figure 8). We also tested a sample of Mobil Artic oil. The Artic
can is clearly older than the other Mobil can¾the logo is older, and there’s no zip code listed with the address, so it’s pre-1963. A straight 20W, it’s labeled as HD oil, meeting “Car Builders’ Most Severe Service Tests.” While it’s “Artic” and not “Arctic,” we can’t help thinking this oil is meant for cold-weather operation. The can even looks like it’s ready for winter: all white, but with a little color on it so you don’t lose it in the snow when you’re out in the tundra changing your oil. This one definitely has an unusual additive package, relying heavily on barium (maybe it’s got a purpose after all!). Interestingly, less zinc is present than phosphorus (Figure 9). Nowadays it’s the other way around.
Phillips 66 Trop-Artic 10W/40
We were going to stop with Mobil Artic, but we can’t resist comparing that one with Phillips Trop-Artic. We’re not exactly sure what Trop-Artic means, but since the can is selling itself as All-Season we’re guessing it’s something along the lines of “use it in the tropics, use it in the arctic.” A 10W/40 in viscosity, this oil looks a lot like what we see out of modern 15W/40s¾a stout additive package and a relatively thick viscosity (Figure 10). In other words, even though this oil is several decades old, it would be fine to use in your F150 tomorrow.
Okay, ten old oils is all we can do for this article. Fortunately the next installment will contain such gems as old versions of Amsoil, Castro, Sinclair, Amoco, Lucky Strike, Union 76, and more! Tune in next time for the next installment of the eBay oils!